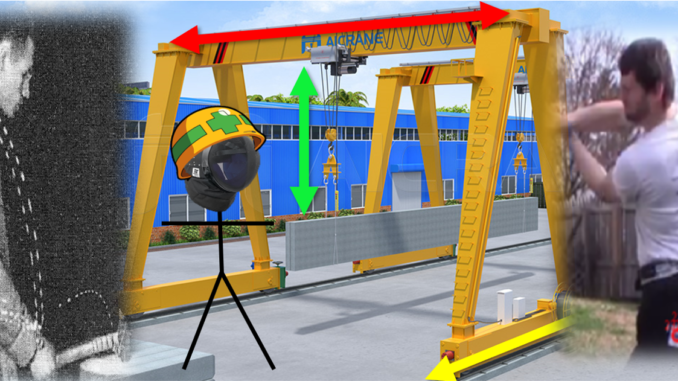
Before we can dive into how to control the body, we need to have an appreciation for just how difficult it is to control the body. We use our bodies every day, so it seems natural and unremarkable. In truth, even the smallest motions are way more complex than you ever appreciated. This is due to the compounding nature of degrees of freedom at every joint in the human body.
This is Part 2 of my series on Ecological Approaches/Psychology. The article stands alone, but is part of a broader modern understanding of human action and perception.
- Part 1: Ecological Psychology
- Part 2: Motor Solutions (you are here)
- Part 3: Affordances
- Part 4: Direct Perception
- Part 5: Perception-Action Coupling
- Part 6: Control Laws
- Part 7: Implications of EA
Degrees of Freedom: Determinate
A degree of freedom is a way in which something can move. The number of degrees of freedom is the number of different ways something can move. If we have a train on a track it has one degree of freedom, it is free to move anywhere along the track but nowhere else. If you ask it to go forward 1km and to the side 500m it is a fundamentally impossible task. In controlling the train you have one degree of freedom at your disposal, and therefore can only act in one dimension.
To move in 3D space we need at least 3 degrees of freedom. A gantry crane is an excellent example of this. In the drawings below we have each axis covered by a single movement element, allowing the crane hook to be located anywhere in the 3D space.
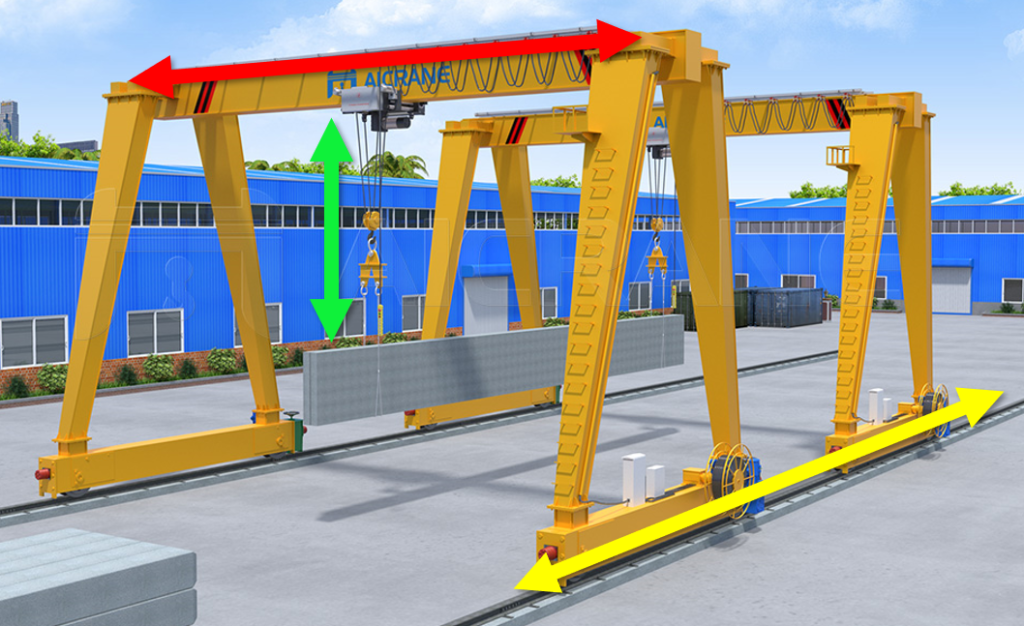
Note that this doesn’t only mean linear movement. Rotation is also a degree of freedom. The gantry crane relies on only linear movement, but a more familiar tower crane uses the exact same concept, just with a rotational degree of freedom substituted in. Three degrees of freedom allows the crane to reach everywhere in 3D space.
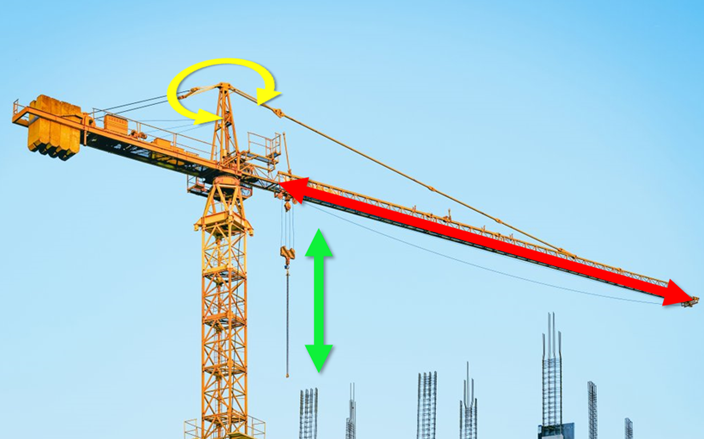
Degrees of Freedom: Indeterminate
You’ll notice that both the crane examples are similar, they use 3 degrees of freedom to move in 3 dimensions of space. This means that for every position in space there is exactly one solution to reach it. If you want to get to point (5,2,3) with a gantry crane you have the x-axis motor take you to x=5, you have the y-axis motor take you to y=2, and you raise/lower the hook to z=3. This is a determinate solution, aka “solvable”.
If you add more degrees of freedom you get an explosion of possible solutions. In the image of a simple robot control arm below we see that it has two joints that are not mutually exclusive in terms of movement. You can not solve for a single solution on how to get from the blue dot to the red dot, there are infinitely many solutions. Two are shown below.
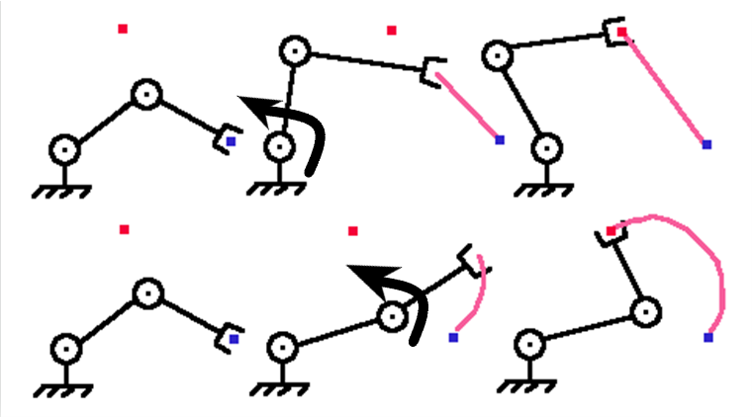
(This image comes from Myles Cupp’s “Why n00bs Fling the Sword: A Multi-Part Analysis of Rapier Thrusting Mechanics” article, which also explores the idea of indeterminate motor solutions for rapier thrusting.)
As you know, in the human body we have more than one joint that can be articulated[citation needed].Which means that anything and everything that we want to do is an indeterminate solution.
Statically Indeterminate: It Gets Worse
So we’ve established that if we have multiple joints moving it gets super messy. It doesn’t end there. What I’ve been describing are indeterminate solutions for the joint angles, but we also have the muscle force activations to think about. Let’s go back to first year engineering school, and use a truss bridge example. The following is a pretty stock problem we would be given to solve.
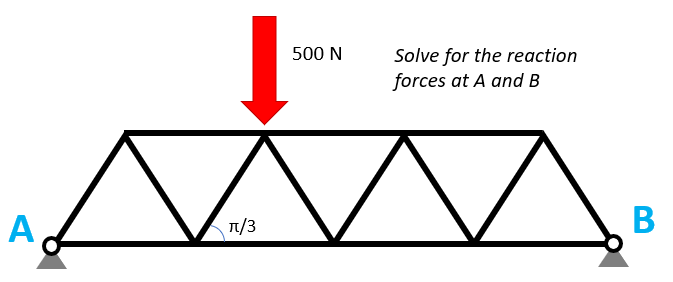
That was pretty straightforward to solve if you know what you are doing, but the details of how you get there are unimportant. Ultimately you have forces from the ground pushing against the truss at A and B, and since the force is closer to A then you get more force on A.
So let’s say the teacher decides to spice it up and adjust the angle of the force at the top. What happens? Well in this case the sadistic teacher returns to the teaching lounge cackling evilly because they have given you a problem that is statically indeterminate: aka completely unsolvable.
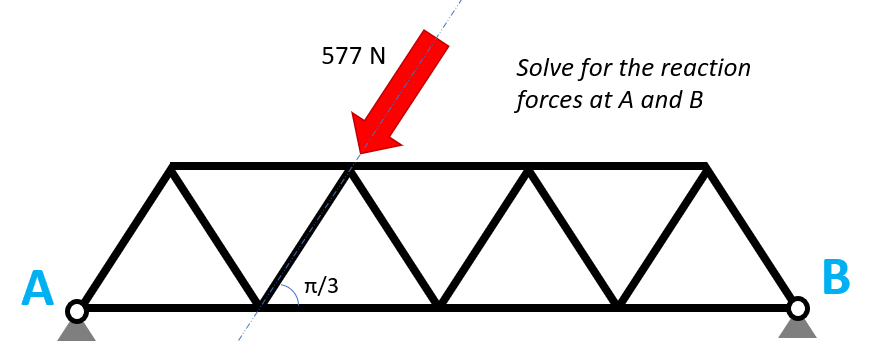
The vertical components of the forces at A and B are identical, and will have the same solution as the first example. However there is still the horizontal force to be taken up by the supports. But how much of this force will each support take? It is impossible to tell! You could have a 50:50 split, you could have 100:0, or you could have 21:79. All solve the problem mathematically, and all are equally valid: the definition of statically indeterminate.
Human Body: Indeterminate Nightmare
Let’s take a look at all of the muscles acting on the shoulder, and the line of action they exert force on.
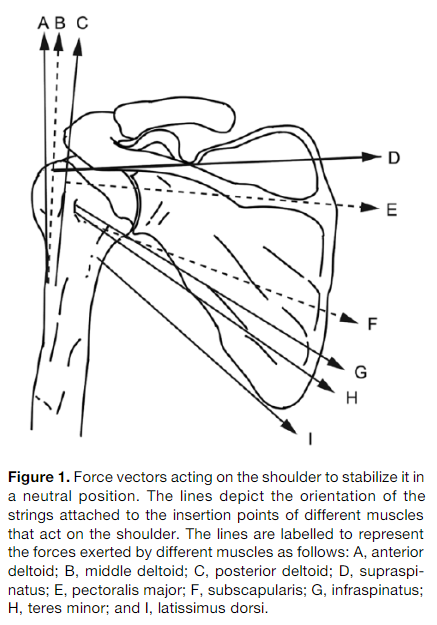
A whopping NINE different force vectors that need to be balanced to accomplish anything! Just to keep your shoulder steady you need an incredible amount of coordination between all the muscles, each adjusting force in harmony to make the overall force balance on the shoulder whatever is needed for the task at hand.
And now we have to account for the fact that we have to solve this problem on all 360 joints at once! (And it is all of the joints at once. Electrography studies of people lifting their arm show activity in the glutes before the shoulder activates, as moving one part of the body recruits entire stabilizing chains through the whole musculoskeletal system.) Which brings us to the core question that both IPA and EA are trying to address:
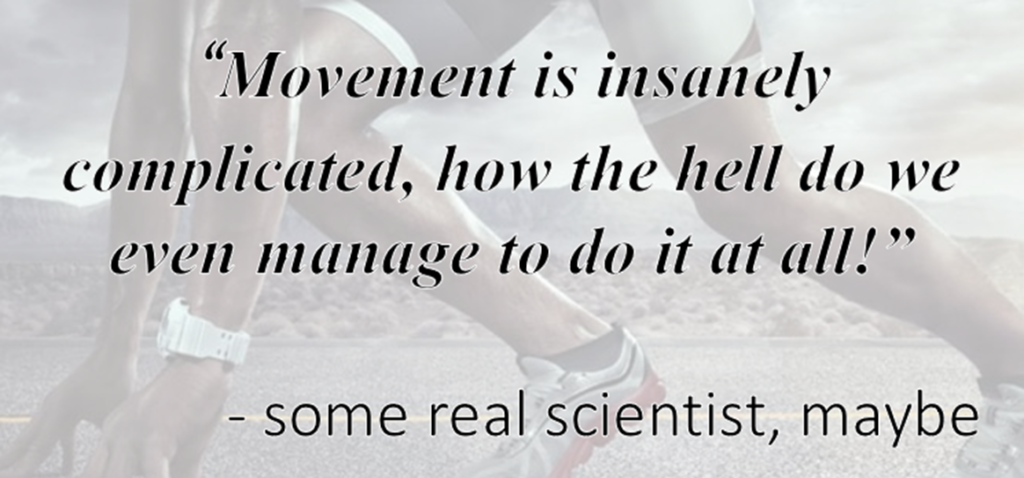
Bernstein Smashes The Established Model
We now go back in time 100 years, to a scientist in the newly established Soviet Union: Nikolai Bernstein. In the 1920s he was tasked with studying blacksmithing, for the purpose of developing ways to improve production efficiency. In the process of these investigations he became one of the first scientists to make heavy use of cyclograms in human motion analysis, a technique in which lights are attached to objects of interest, and their position on each film frame recorded.
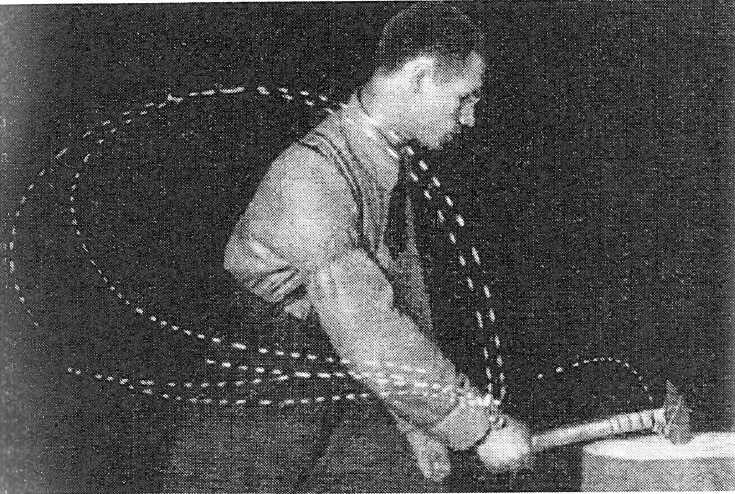
The points were then meticulously measured and the information recorded by hand. This was then scaled and interpreted with the frame rate, to produce tables of the velocity and position of the joint at any given point of time. To my modern computer-addled brain the effort required to do hundreds of trials and calculate every frame by hand is mind boggling.
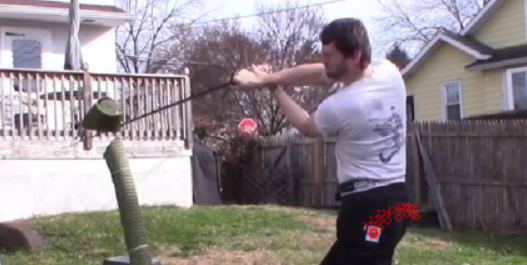
The findings were groundbreaking. It turns out that expert blacksmiths are able to repeatedly hit the target with almost the exact same angle and position every hit, and the non-experts are much worse at it. (Ok, maybe that part was not so interesting).
Based on the IPA model we would expect that the experts are the best because they have efficient motor “programs” in place that take into account all the variables to execute the exact precise motion. But that is not what happened at all. Instead the experts never followed the same motor pattern twice! This observation completely flew in the face of conventional assumptions about how we learned and controlled motion.
Explaining this in a HEMA context, let’s look at a difficult but isolatable skill: performing a double cut on a tatami mat. In a double cut you must cut the bottom of the target, then cut the top before it can move out of position. This is facilitated by having an extremely smooth initial cut, while maintaining form so that a second cut can be executed in quick succession. Per conventional IP thinking in order to be really good you would want to have an extremely well refined program stored for cutting, and deviate from it as little as possible. (The deviation being the error or imperfection that causes the execution to fail.)
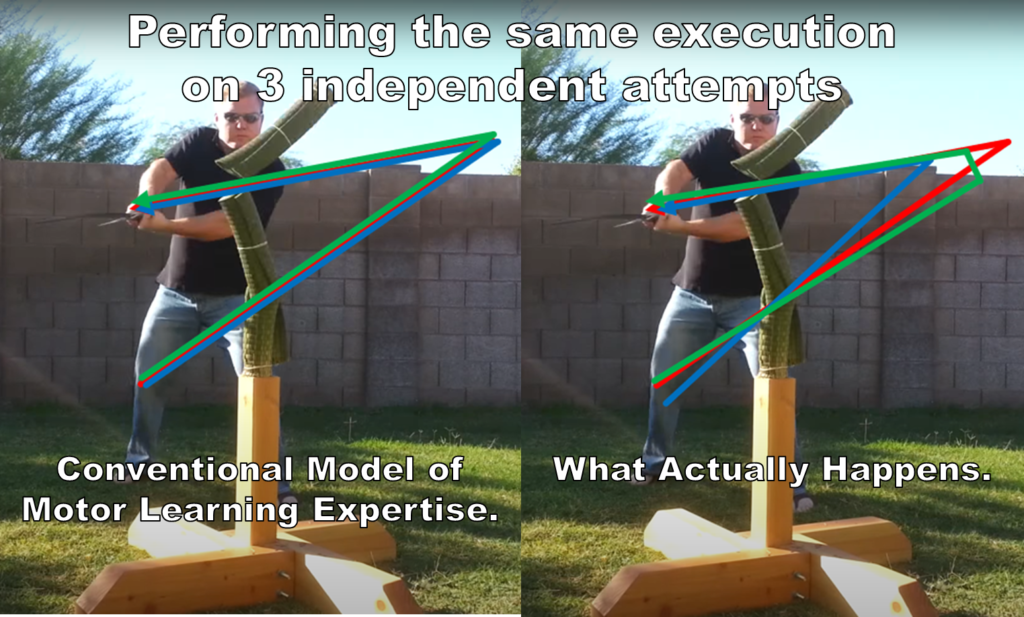
Though I have not done a study based on HEMA (because I’m too busy writing articles like this), based on Bernstein’s studies* on blacksmithing you would actually expect to see that when the skill is repeated it does NOT look the same. Even though the final outcome (the cuts on the mat) are repeatable with a high degree of success, the motor control used to execute it is different. In other words:
“Expertise is not being able to repeatedly generate ‘correct’ motions. Expertise is being able to achieve the correct result no matter the variation in motion.”
*As I stated at the beginning of this series of articles, basing any kind of insight on a single study is a bad idea. In this case I’m using the first breakthrough study that has been since confirmed by other independent investigations.
Repetition Without Repetition
So why does this variability make any sense? In the traditional IPA model any variability in the motion is noise which leads to unpredictability in the final result; precision is achieved through replication of an idealized motor program.
The idea advanced by Bernstein is that rather than figuring out exactly what we need to do ahead of time, the body is constantly taking the information available to it on limb position and sensory perception to be performing continuous online control of the muscles. The expertise we are gaining is the ability to make the adjustments to every muscle as we go through the movement. There have been studies that show experts can have the error decrease through the motion, which is kind of like starting a cut while falling over but still having the final result be good. (Though in the researchers’ case it’s more like measuring slight angle discrepancies over the course of a baseball pitch. I’m guessing a university ethics committee might take issue with a study involving sharp swords and people falling over.)
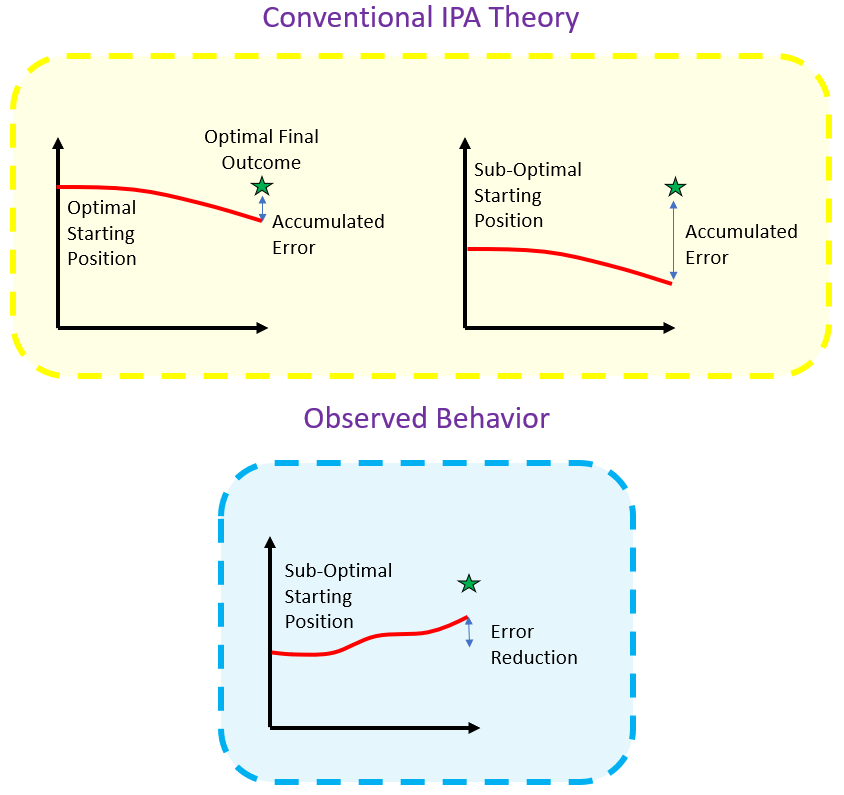
This decrease in error is impossible in a “motor programs” framework. Using this framework the optimal motor solution is loaded and the motion executed, which means any conditions which deviate from expected (slight imbalances in posture, air resistance, muscle fatigue, etc) will make the control worse. As it turns out we are far more resilient, and experts find ways to get the final outcome regardless of the differences in conditions.
Freezing:
As we know from above, doing the online control of all the muscles simultaneously is a big task. In order to simplify the task the body can do something called “freezing”, whereby some of the joints are ‘locked’ and thus don’t have to be factored into the overall movement solution. (Or in math terms, reduce the degrees of freedom of the problem.) This is why beginners are very blocky when performing skills. They are freezing most of their joints and only controlling a handful to achieve the task. As skill in generating the movement solution increases, the other joints will begin to be factored in and the precision and fluidity will increase.
Search for Ideal Form
This idea of a “proper” way to do things permeates all aspects of learning physical skills. As one would expect, people have set out to determine the optimal ways to do different skills. Take one baseball study, where the joint motions of a subsection of professional pitchers was sampled. They took the data to look for the ‘rule’ of how the joints should be timed and found… nothing. By looking at the best players in the world they weren’t able to find what the optimal way to throw a ball is. Which begs the question of how the coaches were able to have an optimal way to teach? 🤔
Does this mean that all ways of doing things are equal? No, of course not. That would be dumb. There are clearly some things that are physical constants which emerge in any method to generate a quality end result. You will generate the most power from the big muscles, and generate the most speed by having the impulse flow successively through from big –> small (aka kinetic chain). These are what are known as “attractors”, the types of things all motion solutions will need to exploit in order to be successful. But what it does mean is that the exact details of how high was the hand, what was the elbow angle when the wrist moved, and such, DO NOT HAVE A “PROPER” POSITION. The optimal way is different for everyone, and as a coach the task is helping their student’s body find a way.
Training Implications
At a superficial read that might just sound like “yeah, I know that the form will be slightly different for everyone depending on their body type”. Which seems pretty obvious and uninteresting. But this is about more than that.
You know that ideal form we spent hours of grueling repetition to get right? It doesn’t exist. There is no “right”. Rather, expert performance comes from being able to figure out how to do a slightly different form based on the circumstances, not repetition of a Platonic form.
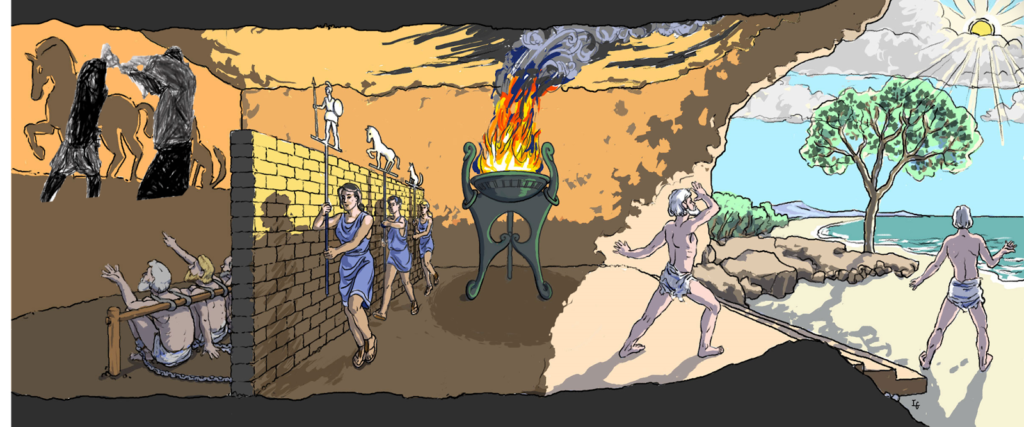
And that is an incredibly radical idea in comparison to the common knowledge of how people get good. (Reminder that links to the best books that break down these ideas and the research in more detail are in the first article.)
Up next: Part 3: Affordances.
What Does This Mean?
I’ll wrap up each article with a more concrete example of what implications the concept has for coaching. In regards to Motor Solutions the most important takeaways are that motions do not need to be taught and directly teaching motions is not the way to generate expert performance.
When you get someone who has never held a sword before you may be tempted to give them a lot of instruction on how to swing or step “correctly” so they don’t generate bad habits. Instead they will learn better fundamentals by just giving a goal from day one. Instead of telling them to keep their hands high and step deeply when cutting you can just hand them a sword and tell them to hit somebody who they will have trouble reaching. They will learn better mechanics faster this way. (And when you need to further refine their abilities, add specific constraints that make their old motor solution non-workable so they need to adapt.)
Stuff For Nerds: Boundary Conditions
When I was doing the truss example I also wanted to mention boundary conditions. But that wasn’t really useful for understanding the types of motor control theories in the human body, so I didn’t. But now that you’re here, I’m going to do it anyway.
The way one frames a problem like this is highly dependent on the boundary conditions. That is, how the element you are studying interacts with the wider world. In the diagram I used, the truss was attached using a “pin” type connector. This means that the connection can support forces across it, but can’t add any torque.
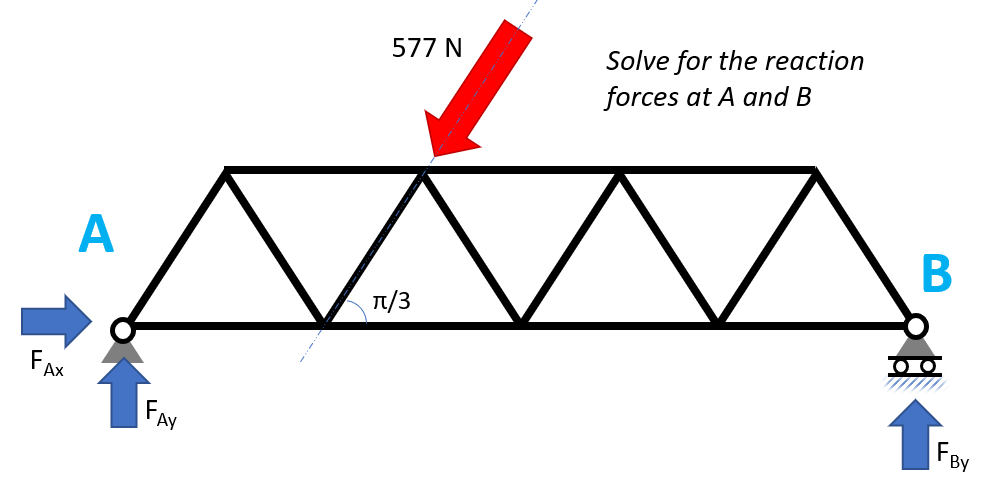
To make this problem solvable we need to eliminate one of the horizontal forces. We can do that by redefining the connection type. Adding a roller to B means that it can no longer support a horizontal force, leaving all of it to A. This now makes the truss solvable, and is something you would see in a first year statics course.
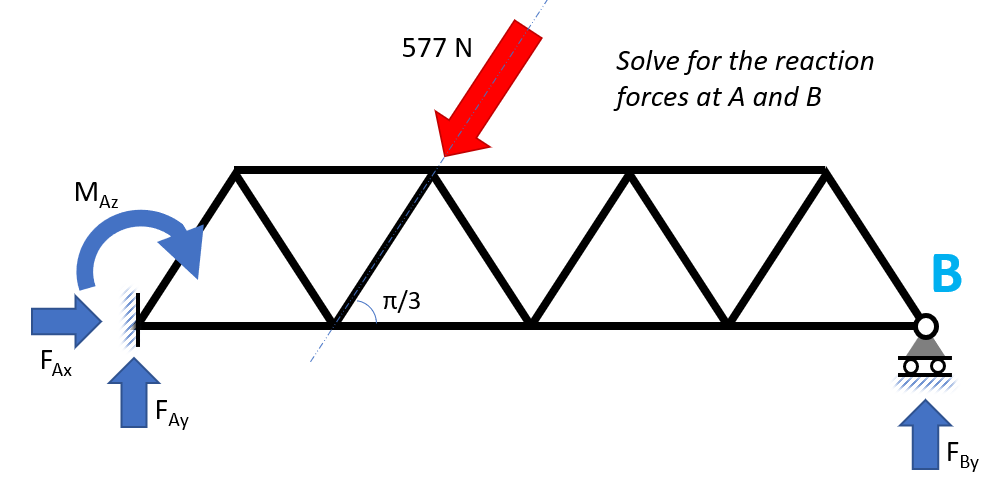
So far I’ve only talked about forces across the connection, but not moments (aka torques). If, instead of pinning A (using a bolt or something) you welded A, it would now be indeterminate again. This is because you don’t know how much of the force holding up B is coming from the support at B, and how much is coming from the weld at A which prevents the truss from rotating. For all you know there is no force at all on B. (That’s one hell of a weld!)
Getting back to motor control, the way something is attached is also going to be a confounding factor. The shoulder has multiple muscles attached at multiple points, at multiple angles, and depending on the initial position of the arm the same muscle might generate arm motion in a different direction.
This is also, somewhat, where the “constraints” in Constraints Lead Approaches come from. Like the forces on the truss are defined by the constraints (aka, connections), the motor solutions of humans will be defined by the constraints of the tasks demanded.
While I hope the main body of this article impressed upon you how difficult a problem motor control is, the rabbit hole goes so much deeper.
(Stuff For Nerds)2
The boundary conditions also make a big difference to sword flex.The following shows how changing the boundary conditions (aka how you hold the sword) has a huge effect on the apparent stiffness of a sword blade.
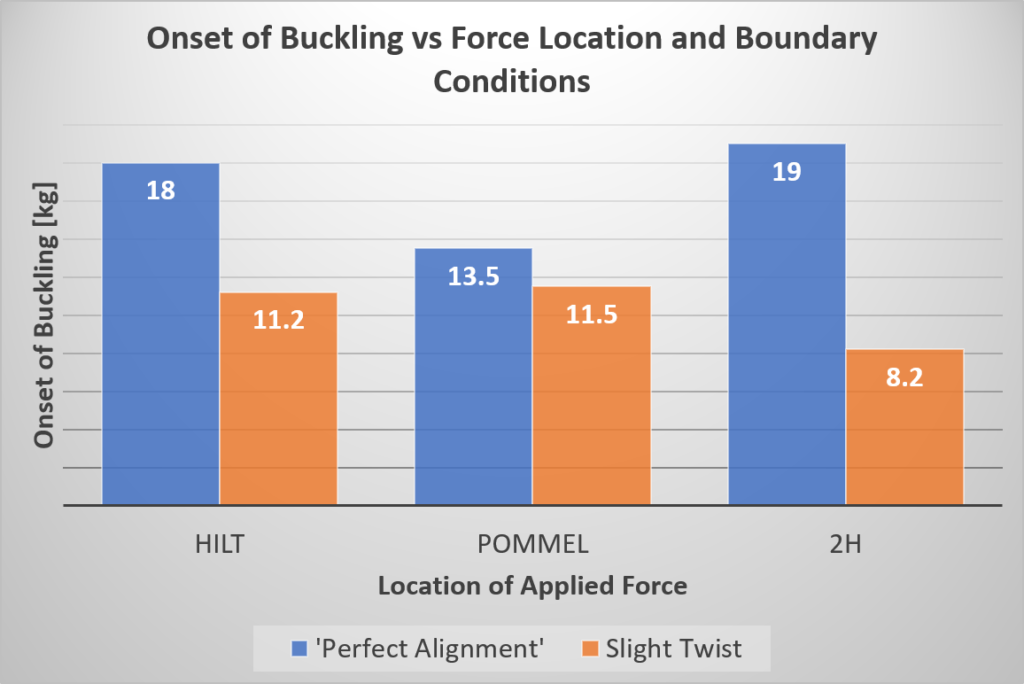
Difficulties with SCA Flex Test and Buckling Test for Measuring Blade Stiffness
And the vibrational behavior!
Analysis of the Vibrational Characteristics of a Training Longsword