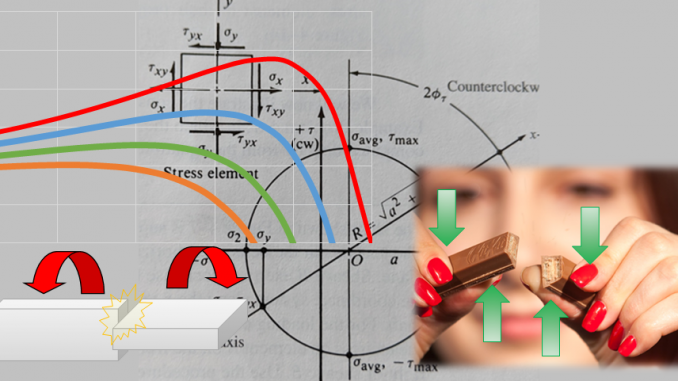
Stress is the force applied, divided by the area on which the force is applied. (What is Force Dispersion? And how it keeps you safe from swords). We normally think of this when examining the effect of a sword on the body. For instance: I can swing a sword at you and have the sword exert a force on your arm when it hits. Depending on the contact surface area:
- Blade is sharp, and the contact surface area is extremely small. Contact stress is extremely high, and the tissue and bone is severed.
- Blade is blunt, and the contact surface area is larger but still small. Contact stress is high, and the tissue is bruised and the bone is broken.
- Protective equipment is worn, which distributes the impact over a large area. Contact stress is low, and there is no damage to the arm.
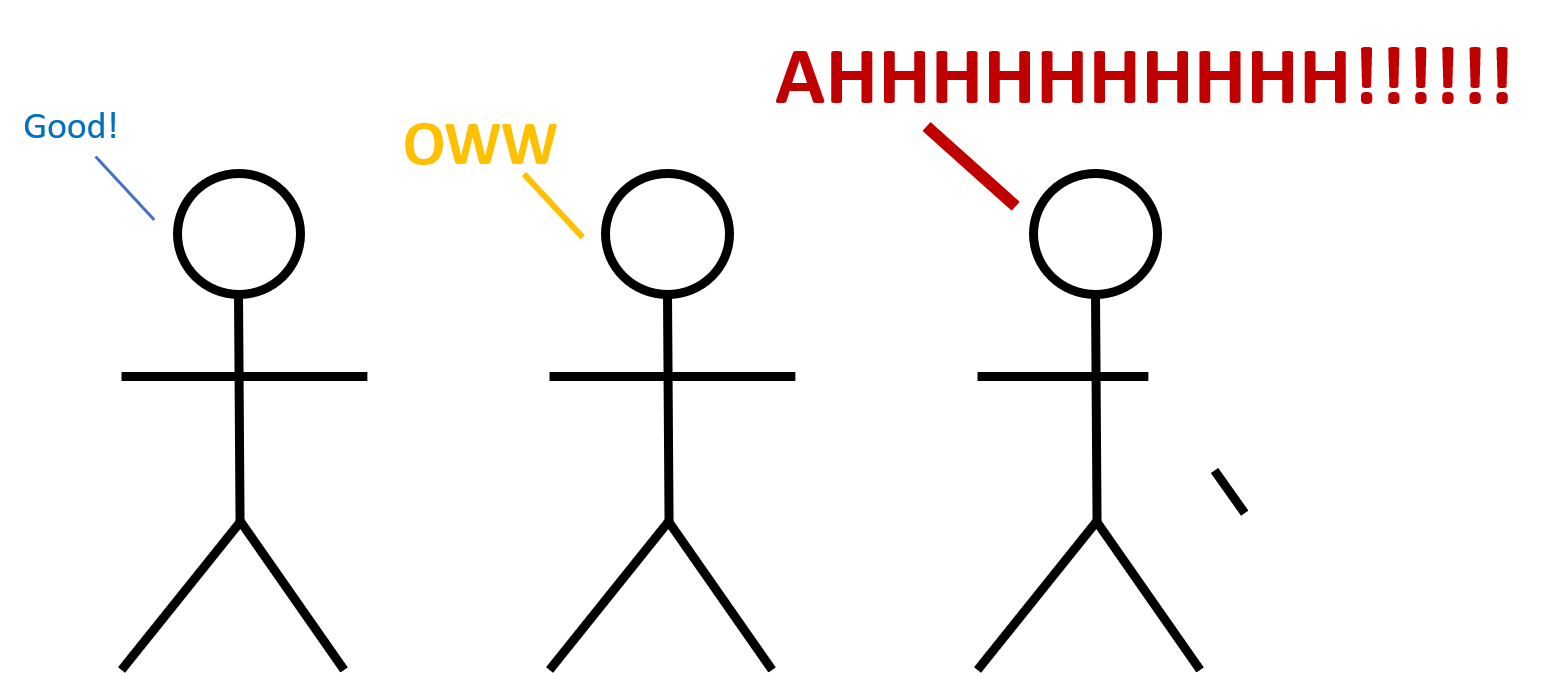
The same mechanics are at play within the sword itself. The force that is being exerted on your body is also being transmitted back into the sword. And rather than being absorbed by weak flesh and bone, the force is acting on hardened steel.
But just because steel is strong, doesn’t mean it is invincible. If you apply a stress on steel beyond its yield stress it will bend plastically (take a set), and if you apply a stress beyond the ultimate stress it will break. But just how much stress is being created in a blade, and where?
Shear Strength
The first type of stress we will look at is shear stress.This is a stress which is applied across the long axis of the blade. The amount of the shear stress is determined by:
- How much force is applied
- The cross sectional area
If you remember anything from grade school geometry it might be that the area of a rectangle is the length times the width. Which means that for a sword the shear stress is the same whether you are applying the force on the edge or on the flat.
The shear force is going to be the same the entire length of the blade, however the cross sectional area is not.
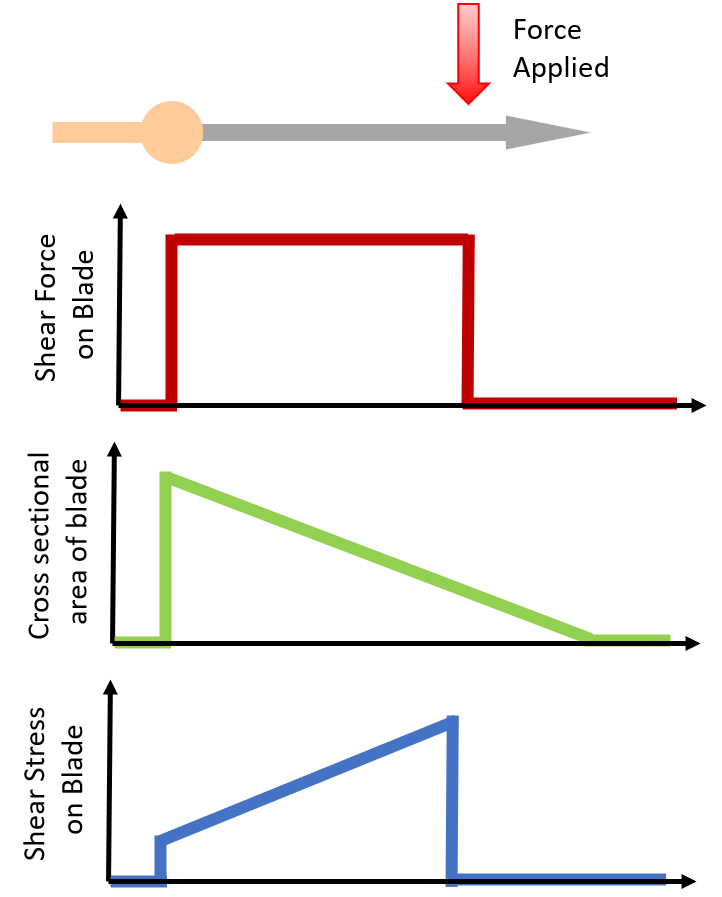
Which means that the maximum shear stress is right on the impact point of the sword.
Axial Loading
Axial loading is force applied directly into the end of a beam. While we do this a lot in swordfighting (aka, stab people), the overall magnitude of the stress it produces on a blade is low in comparison. But keep an eye on the concept, it won’t go away.
Buckling
Buckling is when you load something up in axial compression until it starts to bend. In swords this is ubiquitous. We know and expect all of our blades to have flex, the question is only how much. In structural engineering buckling is regarded as more of a pass/fail criteria. You design so that you will never buckle.
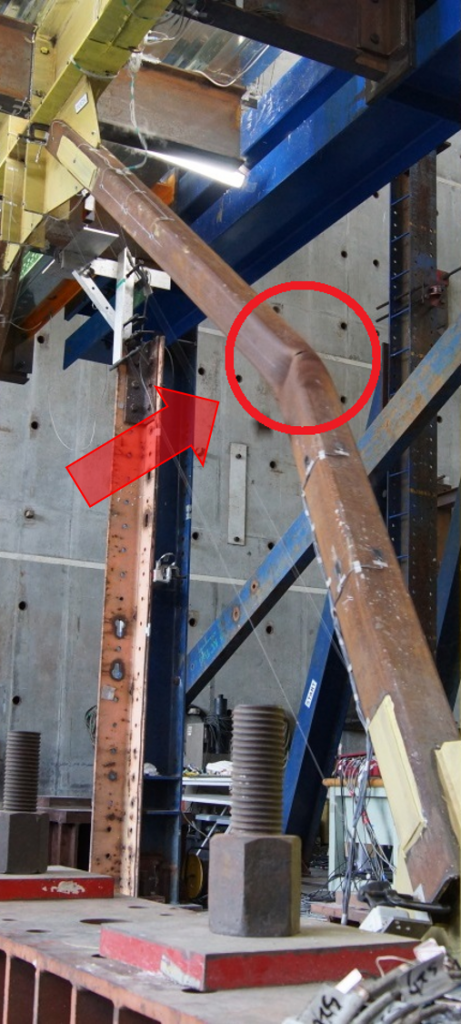
The buckling in the blade produces stress which is similar to beam bending, so let’s skip ahead to that.
Bending Moment
“Moment” is what engineers call torque so that we can feel superior to people who use more pedestrian terminology. It can manifest itself in a few different ways, such as buckling, mid-span loading, and cantilever bending. For our use case of a sword we are interested in cantilever beams.
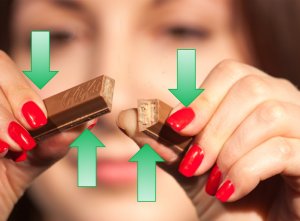
A cantilever beam is one that hangs out and has a force applied to the end. This is the type of loading that you would see on a crane which is lifting a load. There is probably something else we are familiar with that I could use as an example…
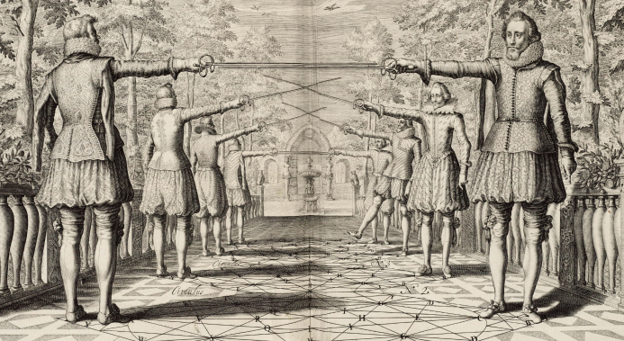
When you hit something with a sword, you are making a nice cantilever beam, applying the load – contact with whatever you are hitting – and the support – your hand(s). This will also generate a bending moment, which is more of a “snap in half” type of behavior, in comparison to the “shear in half”.
When dealing with moment (aka torque) remember it is all force * distance behavior. In the case of the cantilever beam (our sword) this is the distance between the impact point and the part of the blade we are interested in. The actual bending stress is a function of the section modulus, which is a property of the cross sectional shape of the blade. (Learn more about Section Modulus: Do Fullers Make Feders Take a Set?)
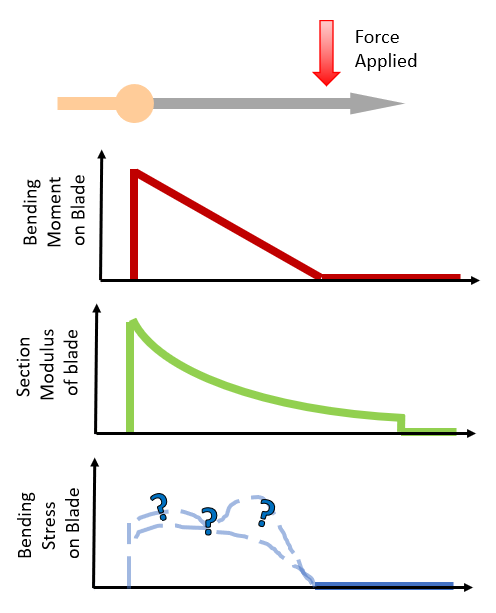
Bending Stress
So why are there question marks in the above plot of bending stress? Because we don’t know what a “typical” blade profile is going to look at in this regard. When looking at the shear stress it is fairly simple. Just about every post-bronze age blade is going to have an increased cross sectional area as it approaches the hilt. So it’s safe to assume that the shear force (which is constant) is going to produce a shear stress which continues to decrease as we approach the hilt.
With bending stress we know that:
- The bending moment increases linearly as we move towards the hilt.
- The sectional modulus increases exponentially as we move towards the hilt.
Now the question is, is there ever a crossover of these points which leads to a higher stress region? It all depends on the blade profile; some swords can create a stress concentration region. This is where the sword is relatively thin, and the bending moment has “overtaken” the section modulus. But later the section modulus “overtakes” the bending moment again, due to its exponential increase.
The impact position is also important. As you bring the impact closer to (or past) this stress concentration point you will see the effect decrease, or go away completely.
Torsional Stress
Torsional stress is the stress you experience from twisting something.
In a straight blade impact we wouldn’t expect to see any torsion. However, how many sword impacts happen perfectly straight? If the blade doesn’t meet the target perfectly in line you will cause torsion. This is what you will see if you cut into a person (or sword) without perfect edge alignment.
Much like shear force, torsional force is constant over the entire blade. However the resistance to torsion, the polar section modulus, is exponentially increasing the closer you get to the hilt. Which means the torsional stress is going to be at its maximum at the impact point.
Force Stackup
When you thrust something with your sword you will see:
- Axial Stress – constant through the whole blade
- Bending Stress – maximum stress point depends on blade profile.
When you impact something along the length of your blade (cut or parry) you will see:
- Shear Stress – highest at point of contact
- Bending Stress – maximum stress point depends on blade profile.
- Torsional Stress – highest at point of contact. Magnitude depends on geometry of impact.
And the resultant maximum stress on the steel is a combination of all forces stacked up together. For example if we combine the shear and torsion forces together:
And if we want to factor all three together?
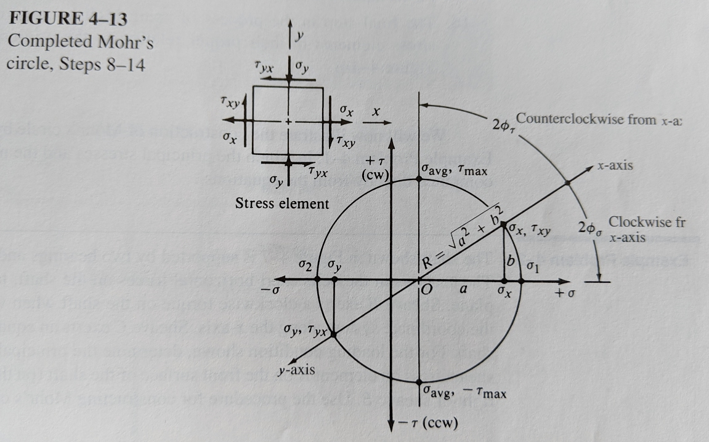
Suffice to say it gets complicated. But for now the takeaways are:
- In most cases the highest stress region of the sword is the impact point.
- For certain blade geometries we could create a maximum bending stress region mid-blade.
- Taking impacts without your edge in-line greatly increases the stress on the blade.